The alignment of a microscope objective and sample demands precise and rapid movements. Based on the inverse piezoelectric effect, products by piezosystem jena offer unique technical characteristics compared to other solutions on the market. They are characterized by almost unlimited refinement of motion while avoiding mechanical play. Thus, they are completely resistant to internal friction.
piezosystem jena has long served OEMs and partners in the microscopy industry by consistently creating and adapting piezo actuators for specific customer needs.
The piezosystem jena MIPOS objective positioners provide up to 600 microns of z-motion with sub-nm resolution. The movement of the MIPOS is characterized by its precisely parallel movement within the optical axis. Additionally, these objective positioners can accommodate lenses from all of the most popular manufacturers including:
- Zeiss
- Nikon
- Leica
- Olympus
A unique feature of MIPOS objective positioners is their extremely high stiffness. This allows for them to be configured for inverted actuation.
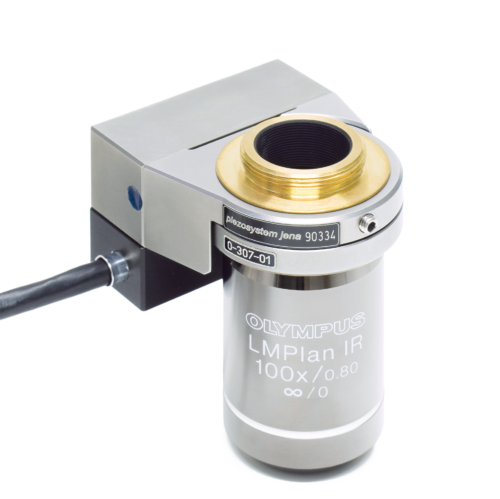
MIPOS Objective Positioner
To move an objective in more than just the z-direction, a 3-axis solution for you in the form of our TRITOR® 102, a 3-axis stage with available threading for your objective. The TRITOR® 102 provides up to 100 microns of motion in x, y, and z, all with nanometer resolution.
Lastly, if you are looking to move your sample rather than your objective, then we have a wide variety of stages that can accomplish whatever your needs may be. Our stages can achieve up to 5 axes of motion, can come with central apertures for inverted microscopy, and can be used in vacuum or cyrogenic conditions.
MIPOS piezo positioners can be used with:
- Simple Microscopes
- Scanning Electron Microscopes (SEM)
- Confocal Microscopes
- Inverted Microscopes
- STED Microscopy
- Fluorescence Microscopy
- STORM Imaging
- Atomic Force Microscopes (AFM)
- White Light Interferometry
Whitepaper: Beyond Microscopy – MIPOS in Industry Applications
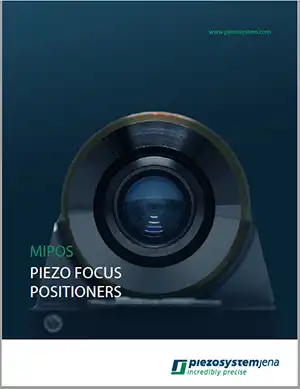